What Is Cupronickel?
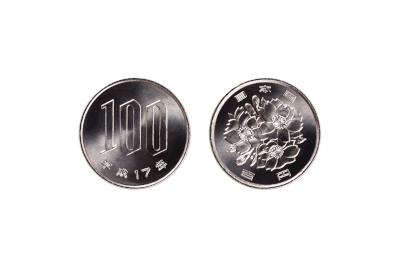
Cupronickel is a copper-based alloy containing nickel and other metals. It is used in a wide variety of applications because of its excellent properties such as corrosion resistance, workability, strength, hardness, castability, and beautiful color. It is also known as copper-nickel.
Because of its good electrical conductivity and corrosion resistance, it is used for electrical wiring. It is also used for musical instruments due to its good castability, and is widely used especially for brass instruments. Its beautiful color and high corrosion resistance also make it suitable for manufacturing coins and medals.
Uses of Cupronickel
1. Electrical and Electronic Components
Wiring, connectors, circuit boards, electrical resistors, coils, transformers, etc.
2. Electric Circuits
High-frequency circuits, RFID antennas, etc.
RFID antennas are antennas used in RFID (Radio Frequency Identification) technology to transmit and receive information at radio frequencies.
3. Automobiles
Radiators, clutch components (e.g., clutch discs), battery terminals, etc.
A clutch disc is part of the clutch mechanism that connects the engine and transmission (the mechanism used to transfer engine power to the wheels) in vehicles such as cars and motorcycles.
4. Architecture
Roofs, exterior walls, door knobs, and metal fences.
Steam turbines, air conditioning units, and freezers.
6. Medical Equipment
Surgical instruments, artificial joints, dental tools, etc.
7. Metal Parts
Screws, washers, springs, gears, shafts, etc.
8. Decoration
Clocks, light fixtures, metal ornaments, etc.
9. Sporting Goods
Golf clubs, tennis rackets, motorcycle frames, etc.
Types of Cupronickel
There are two types of Cupronickel, C7060 and C7150.
Properties of Cupronickel
1. Color
Cupronickel is an alloy of copper and zinc. Since copper and zinc are both metals, cupronickel is a material with metallic luster. Cupronickel has a beautiful white color because zinc can be added to whiten the color of copper, which has a reddish color in its pure state. Since the surface is not easily oxidized, the luster can be maintained for a long period of time.
2. Workability
Cupronickel has good workability. As an alloy of copper and zinc, it is soft and easy to work. It also has a uniform microstructure that evenly distributes stress generated during machining and improves workability.
Furthermore, its relatively low melting point and boiling point allow it to be easily heated and reshaped, making it suitable for use in a variety of processing methods, including casting, forging, machining, and cutting.
3. Corrosion Resistance
Cupronickel has excellent corrosion resistance. The reason is due to the following factors.
First, when zinc is oxidized, it forms an oxide film, which enhances the protective action of copper against corrosion. In addition, zinc acts as a cathode in cupronickel alloys, preventing oxidation reactions and providing electrochemical protection.
Finally, its excellent corrosion resistance is also due to its high stability in water and its resistance to corrosion in seawater and fresh water.
4. Thermal Conductivity
Cupronickel is a metal that generally has high thermal conductivity, which allows it to transfer heat efficiently. It also contains zinc, which is a higher thermal conductor than copper, so the overall thermal conductivity is higher when cupronickel contains zinc.
The crystalline structure of cupronickel is characterized by a random arrangement of densely arranged spherical particles, which makes it a good heat conductor. These factors combine to give cupronickel excellent thermal conductivity.
5. Electrical Conductivity
As a metal, cupronickel generally has high electrical conductivity, which allows it to transfer electricity efficiently. In addition, the zinc in cupronickel has a higher electrical conductivity than copper, which helps to increase the overall electrical conductivity.
Furthermore, cupronickel has a densely packed crystal structure, which allows electrons to move freely, resulting in higher electrical conductivity.
6. Strength, Wear Resistance
Cupronickel, an alloy of copper and zinc, has a good balance of hardness and flexibility, and has high strength and excellent wear resistance. It also has a uniform microstructure, with fine crystal particles randomly arranged. Therefore, it distributes stress evenly and improves strength, while at the same time picking up fine crystal particles wear substances.
Specifically, when wear materials created by friction adhere to the surface of cupronickel, the crystalline particles encapsulate the wear materials and prevent their progression. The fine crystalline particles pick up the wear material, making cupronickel highly resistant to wear.
Cupronickel has a smooth surface and generates less heat due to friction, resulting in higher wear resistance.
Other Information on Cupronickel
Magnetism of Cupronickel
Copper is weakly paramagnetic under normal temperature and pressure, and zinc is also weakly paramagnetic under normal temperature and pressure, but cupronickel, an alloy of the two, is a non-magnetic material.
This is closely related to cupronickel’s high electrical conductivity. When a metal is exposed to electromagnetic waves, a magnetic field is generated, and this magnetic field can generate an electric current inside the metal (electromagnetic interference), which can be a problem in high-frequency circuits, for example.
Cupronickel, however, does not generate a magnetic field and does not cause electromagnetic interference, making it suitable for use in electric circuit components such as high-frequency circuits.